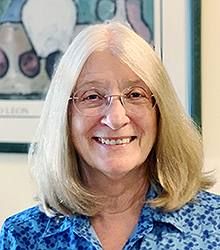
Dr. Moody received her bachelor’s degree from Goucher College. She conducted her MS research in neuroscience under the tutelage of Richard Meszler, PhD, at the University of Maryland School of Dentistry, which launched her career path exploring the nervous system. She earned her PhD in neuroscience from the University of Florida and served a postdoctoral fellowship at the University of Utah until 1983. She then went to the University of Virginia and served on the faculty of the Anatomy & Cell Biology Department, the Department of Neuroscience, and the Developmental Biology program. In 1992, Dr. Moody joined GW as a full professor in the Department of Anatomy & Cell Biology.
For more than 40 years, Dr. Moody’s lab has been working on issues specific to the development of the nervous system including the cranial sensory structures. Her research aims to discover mechanisms regulating neural cell fate and causes of birth defects.
Dr. Moody has published more that 100 scientific articles, numerous book chapters and reviews and five books, co-edited the “Evolutionary Cell Biology” book series, served on several scientific advisory boards, editorial boards and professional society executive boards. She currently is the Special Contents editor for the journal “Developmental Biology”. Dr. Moody has received grants for her research from the National Institutes of Health, the National Science Foundation, the US-Israel Binational Science Foundation and the March of Dimes. She has been recognized for her contributions by being elected a Fellow of the American Association for Anatomy, a member of the Society for Developmental Biology Academy, and receiving the Lifetime Achievement Award from the International Xenopus Conference and the David W. Bixler Distinguished Scientist Award from the Society for Craniofacial Genetics and Developmental Biology.
- Lab Members
-
Past Lab Members
- Tammy Awtry, PhD, Science Communicator, Cure Alzheimer’s Fund
- Daniel Bauer, MD, PhD, Internal Medicine, Physician Associates of Virginia
- Aparna Baxi, PhD, Postdoctoral fellow, NIH-NIDCR
- Samantha Brugmann, PhD, Professor, Cincinnati Children’s Hospital Medical Center
- Zoya Demidenko, PhD, HRI Scientist, Roswell Park Cancer Institute
- Kathleen E. Dennis, PhD, Research Assistant Professor, Vanderbilt University
- Betty C. Gallagher, PhD, Research Scientist, University of Virginia (deceased)
- Stephen Gee, PhD, Assistant Professor, Worcester State University
- Paaqua Grant, PhD, Management and Program Analyst, US Department of Veterans Affairs
- Alexandra Hainski-Brousseau, PhD, Science Educator
- Sen Huang, MD, PhD, Director, Huang Clinic, Washington DC (retired)
- Karyn Jourdeuil, PhD, Staff Scientist, NIH-NIDCD
- Stephanie Keer, PhD, Assistant Professor, Lincoln Memorial University
- Kristy L. Kenyon, PhD, Professor, Hobart and William Smith Colleges
- Kathryn B. Moore, PhD, Professor, University of Utah
- Zahra Motahari, PhD, Project Scientist, University of California, Irvine
- Karen M. Neilson, PhD, Program Director, NIH-NHLBI
- Petra D. Pandur, PhD, Training and Coaching Manager, Leadership Sculptor, Gmbh
- Ankita Shah, PhD, Global Market Development Program, AbbVie Pharmaceuticals
- Steven A. Sullivan, PhD, Senior Research Scientist, New York University Center for Genomics and Systems Biology
- Andre L. P. Tavares, PhD, Assistant Professor, University of Delaware
- Bo Yan, MD, PhD, Director, Translational Medical Research, Jining Medical University
- Norann Zaghloul, PhD, Program Director, NIH-NIDDK
- Research
-
The Moody laboratory studies two aspects of neural developmental gene regulatory networks: (1) molecular mechanisms by which early ectodermal genes regulate the formation of the epidermis, neural border zone (neural crest and cranial placodes) and the neural plate; and (2) novel co-factors and down-stream targets of the Six1 transcription factor, a key regulatory gene that specifies placode-derived sensory structures of the vertebrate head. This information is being used to discover new genes involved in neural tube and craniofacial birth defects.
The Role of Maternal Determinant Molecules in Establishing Neural Fates
Using cell lineage analyses, single blastomere transplantation and culture, PCR analyses and gene mis-expression, we showed that dorsal-animal maternal molecules bias one side of the embryo to acquire a dorsal fate shortly after fertilization. Dorsal axis-inducing activity can be activated by an activin-like signal and by localized polyadenylation between the 8- and 16-cell stages. Ventrally localized Wnt8b signaling antagonizes this dorsal-axis activity. Noggin signaling from animal blastomeres promotes a neural fate in vegetal equatorial cells. We identified 40 unique mRNAs that are enriched in the animal blastomeres using a microarray approach. We are functionally characterizing a number of these to discover whether they bias cells towards a neural fate. Recently in collaboration with Dr. Peter Nemes (GWU Chemistry) we used Mass Spectrometry to identified metabolites and proteins that are unique to specific blastomeres of different fates.
We cloned two maternal genes (foxD4, flotillin1) and studied their functions in establishing neural fate in dorsal, animal lineages. For a description of FoxD4, please see: A gene regulatory network involved in neural plate development.
Flotillin1 is a member of a family of membrane-associated proteins that are highly expressed in the nervous system. These proteins are highly enriched in growing axons. There are three Xenopus alleles (flotillin 1a, 1b, 1c) that have very similar expression profiles. Flotillin1 is expressed during oogenesis and transcripts become enriched in the animal hemisphere precursors of the embryonic ectoderm. Zygotic transcripts are expressed in the presumptive neural plate, with lower levels in the non-neural ectoderm. At neural tube closure, Flotillin1 is expressed in the entire telencephalon, dorsal domains of the rest of the neural tube and dorsal paraxial mesoderm. Primary sensory neurons (Rohon-Beard cells) express Flotillin1 at particularly high levels. At tail bud stages, placodal and branchial arch structures additionally express Flotillin1. While flotillin1 has been implicated in axon regeneration, its function during early development is not known.
-
Related Articles
-
- Neal, S.J., Rajasekaran, A., Jusic, N., Taylor, L., Read, M., Alfandari, D., Pignoni, F. and S.A. Moody (2023) Using Xenopus to discover new genes involved in congenital hearing loss syndromes. J. Experimental Zoology, Part B. doi.org/10.1002/jez.b.23222.
- Onjiko, R.M., P. Nemes and S.A. Moody (2021) Altering metabolite distribution at Xenopus cleavage stages affects left-right gene expression asymmetries. genesis, The Journal of Genetics and Development, 59: e23418. doi: 10.1002/dvg.23418. PMID: 33826226.
- Moody, S.A. and S.L. Klein (2019) Deferred-use molecules and decision-making in development. In: Deferring development: Setting aside cells for future use in development and evolution. C. Bishop and B.K. Hall Eds., CRC Press, pp 29-52.
- Lombard-Banek, C., S.A.Moody, C. Manzini and P. Nemes (2019) Microsampling capillary electrophoresis mass spectrometry enables single-cell proteomics in complex tissues: Developing cell clones in live Xenopus laevis and zebrafish embryos. Analytical Chemistry 91: 4797-4805. doi: 10.1021/acs.analchem.9b00345. PMID: 30827088.
- Sullivan, C.H., H.D. Majumdar, K.M. Neilson and S.A. Moody (2019) Six1 and Irx1 have reciprocal interactions during cranial placode and otic vesicle formation. Developmental Biology 446: 68-79. doi: 10.1016/j.ydbio.2018.12.003. PMID: 30529252.
- Baxi, A., C. Lombard-Banek, S.A. Moody and P. Nemes (2018) Proteomic characterization of the neural ectoderm fated cell clones in the Xenopus laevis embryo by high-resolution mass spectrometry. ACS Chemical Neuroscience 9: 2064-2073. doi: 10.1021/acschemneuro.7b00525. PMID: 29578674.
- Onjiko, R., E. Portero, S.A. Moody and P. Nemes (2017) In situ microprobe capillary electrophoresis mass spectrometry: metabolic reorganization in single differentiating cells in the live vertebrate (X. laevis) embryo. Analytical Chemistry 89: 7069-7076. PMID: 28434226 (Cover article)
- Onjiko, R., D.O. Plotnick, S.A. Moody and P. Nemes (2017) Metabolic comparison of dorsal versus ventral cells directly in the live 8-cell frog embryo by microprobe single-cell CE-ESI-MS. Analytical Methods 9: 4964-4970. PMID: 29062391.
- Onjiko, R.M., E.P. Potero, S.A. Moody and P. Nemes (2017) Microprobe capillary electrophoresis mass spectrometry for single-cell metabolomics in live frog (Xenopus laevis) embryos. J. Vis. Experiments 130: e56956, doi:10.3791/56956. PMID:29286491.
- Lombard-Banek, C., Moody, S.A., and P. Nemes (2016) Single-cell mass spectrometry for discovery proteomics: quantifying translational cell heterogeneity in the 16-cell frog (Xenopus) embryo. Angewandte Chemie International Edition 55: 2454-2458. PMID: 26756663. Link
- Onjiko, R., Morris, S., Moody, S.A. and P. Nemes (2016) Single-cell mass spectrometry with multi-solvent extraction identified metabolic differences between left and right blastomeres in the 8-cell frog (Xenopus) embryo. Analyst 141: 3648-3656. PMID: 27004603. Link
- Gaur, S., Mandelbaum, M., Herold, M., Majumdar, H.D., Neilson, K.M., Maynard, T.M., Mood, K., Daar, I.O. and S.A. Moody (2016) Neural transcription factors bias cleavage stage blastomeres to give rise to neural ectoderm. genesis, The Journal of Genetics and Development 54: 334-349. PMID: 27092474. Link
- Lombard-Banek, C., Reddi, S., Moody, S.A., and P. Nemes (2016) Label-free quantification of proteins in single embryonic cells by capillary electrophoresis high-resolution mass spectrometry. Molec. Cell. Proteomics Jun 17. pii: mcp.M115.057760. [Epub ahead of print]. PMID: 27317400. Link
- Onjiko, R.M., Moody, S.A., and P. Nemes (2015) Single-cell mass spectrometry reveals small molecules that affect cell fates in the 16-cell embryo. Proceedings of the National Academy of Sciences (USA) 112: 6545-6550. PMID: 25941375. Link
- Shrestha, B., Sripadi, P., Reschke, B.R., Henderson, H.D., Powell, M.J., Moody, S.A., and A. Vertes (2014) Subcellular metabolite and lipid analysis of Xenopus laevis eggs by LAESI mass spectroscopy. PLoS ONE 9(12):e115173. PMID: 25506922. Link
- Grant, P.A., Yan, B., Johnson, M.A., Johnson, D.L.E., and S.A. Moody (2014). Novel animal pole-enriched maternal mRNAs are preferentially expressed in neural ectoderm. Developmental Dynamics. 243:478-496 Link.
- Grant, P.A., M.B. Herold and S.A. Moody (2013) Blastomere explants to test for cell fate commitment during embryonic development. Journal of Visualized Experiments (71) e4458, doi: 10.3791/44584. Link
- Huang, H., B. Yan, S.A. Sullivan and S.A. Moody (2007). Noggin signaling from Xenopus animal blastomere lineages promotes a neural fate in neighboring vegetal blastomere lineages. Developmental Dynamics 236: 171-183. Link
- Pandur, P.D., S.A. Sullivan and S.A. Moody (2002) Multiple maternal influences on dorsal-ventral fate in Xenopus animal blastomeres. Developmental Dynamics 225:581-587. Link
- Hainski, A.M. and S.A. Moody (1996) An activin-like signal activates a dorsal-specifying RNA between the 8- and 16-cell stages of Xenopus. Developmental Genetics 19: 210-221. Link
- Hainski, A. M. and S.A. Moody. (1992) Xenopus maternal RNAs from a dorsal animal blastomere induce a secondary axis in host embryos. Development 116: 347-355. Link
- Gallagher, B.C., A.M. Hainski and S.A. Moody. (1991) Autonomous differentiation of dorsal axial structures from an animal cap cleavage stage blastomere in Xenopus. Development 112: 1103-1114. Link
A Gene Regulatory Network Involved in Neural Plate Development
In a screen designed to identify animal localized maternal mRNAs (see: "The role of maternal determinant molecules in establishing neural fates" above), we cloned foxD4 (aka foxD5), which is a member of the forkhead/winged helix transcription factor family. FoxD4 is expressed maternally during oogenesis, and maternal transcripts are confined to the animal hemisphere precursors of the embryonic ectoderm. In over-expression and animal cap assays, it activates dorsal axis genes, both neural and muscle, and induces a secondary axis. FoxD4 also is expressed zygotically in the presumptive neural ectoderm and neural plate. It is induced strongly by Siamois and Cerberus, but only weakly by neural inducers (Noggin, Chordin). Over-expression assays show that FoxD4 expands Sox2+ neural plate stem cells and represses neural differentiation genes. Its expression is down-regulated as the neural tube closes and differentiation begins. We demonstrated that foxD4 acts upstream of numerous co-expressed neural plate genes, some of which stabilize a neural ectodermal fate and some of which initiate neural differentiation. Together these genes define a regulatory network that establishes the neural ectoderm and controls the onset of neural differentiation. We recently showed that the mouse homologue plays a similar role in the transition of pluripotent stem cells to neural progenitors.
-
Related Articles
-
- Marchak, A., K.M. Neilson, H.D. Majumdar, S.L. Klein and S.A. Moody (2023) The sulfotransferase XB5850668.L is required to apportion embryonic ectodermal domains. Developmental Dynamics doi: 10.1002/dvdy.648. PMID: 37597164
- Klein, S.L., A.L.P. Tavares, M. Peterson, C.H. Sullivan and S.A. Moody (2022) Repressive interactions between transcription factors separate different ectodermal domains. Frontiers in Cell and Developmental Biology 10: 786052. doi: 10.3389/fcell.2022.786052. PMID: 35198557. PMCID: PMC8859430
- Baxi, A., C. Lombard-Banek, S.A. Moody and P. Nemes (2018) Proteomic characterization of the neural ectoderm fated cell clones in the Xenopus laevis embryo by high-resolution mass spectrometry. ACS Chemical Neuroscience 9: 2064-2073. doi: 10.1021/acschemneuro.7b00525. PMID: 29578674.
- Marchak, A., P. Grant, K.M. Neilson, H.D. Majumdar, S. Yaklichkin, D. Johnson and S.A. Moody (2017). Wbp2nl has a developmental role in establishing neural and non-neural ectodermal fates. Developmental Biology 429: 213-224. PMID: 28663133.
- Sherman, J.H., Karpinski, B., Fralish, M., Cappuzzo, J., Dhindsa, D., Thal, A. Moody, S.A., LaMantia, A.S., and T.M. Maynard (2017) Foxd4 is essential for establishing neural cell fate and for neuronal differentiation. genesis, The Journal of Genetics and Development, Mar 18. doi: 10.1002/dvg.23031. [Epub ahead of print]. PMID: 28316121
- Gaur, S., Mandelbaum, M., Herold, M., Majumdar, H.D., Neilson, K.M., Maynard, T.M., Mood, K., Daar, I.O. and S.A. Moody (2016) Neural transcription factors bias cleavage stage blastomeres to give rise to neural ectoderm. genesis, The Journal of Genetics and Development 54: 334-349. PMID: 27092474
- Lee, H.-K., Lee, H.-S. and S.A. Moody (2014) “Neural transcription factors: from embryos to neural stem cells” Molecules and Cells 37: 705-712. PMCID: PMC4213760. Link
- Klein, S.L. and S.A. Moody (2015) Early neural ectodermal genes are activated by Siamois and Twin during blastula stages. genesis, The Journal of Genetics and Development 53: 308-320. PMID: 25892704. Link
- Klein, S.L., K.M. Neilson, J. Orban, S. Yaklichkin, J. Hoffbauer, K. Mood, I.O. Daar and S.A. Moody (2013). Conserved structural domains in FoxD4L1, a neural forkhead box transcription factor, are required to repress or activate target genes. PLoS One 10.1371/journal.pone.0061845. Link.
- Moody, S.A., S. L. Klein, B. A. Karpinski, T. M. Maynard and A.-S. LaMantia (2013) “On becoming neural: what the embryo can tell us about differentiating neural stem cells.” Amer. J. Stem Cells 2: 74-94. Link
- Neilson, K.M., S.L. Klein, P. Mhaske, K. Mood, I.O. Daar and S.A. Moody. (2012) Specific domains of FoxD4/5 activate and repress neural transcription factor genes to control the progression of immature neural ectoderm to differentiating neural plate. Developmental Biology 365: 363-375. Link
- Yan, B., K.M. Neilson and S.A. Moody. (2010) Microarray identification of novel downstream targets of FoxD5, a critical component of the neural ectodermal transcriptional network. Developmental Dynamics 239: 3467-3480. Link
- Yan, B., K.M. Neilson and S.A. Moody. (2009) Notch signaling downstream of foxD5 promotes neural ectodermal transcription factors that repress neural differentiation. Developmental Dynamics 238: 1358-1365. Link
- Yan, B., K.M. Neilson and S.A. Moody. (2009) FoxD5 plays a critical upstream role in regulating neural fate and onset of differentiation. Developmental Biology 329: 80-95. Link
- Rogers, C.D., S.A. Moody and E.S. Casey (2009) “Neural induction and factors that stabilize a neural fate” Birth Defects Research part C: Embryo Today 87: 249-262. Link
- Sullivan, S.A., L. Akers and S.A. Moody (2001). foxD5a, a Xenopus winged helix gene, maintains an immature neural ectoderm via transcriptional repression that is dependent upon the C-terminal domain. Developmental Biology 232: 439-457. Link
Specification of Cranial Placode Ectoderm
Placodes are ectodermal specializations in the vertebrate head that contribute to each of the sensory systems (olfactory epithelium, lens, vestibular-acoustic organs and ganglia, cranial ganglia and lateral line). We cloned a member of the Six gene family (Six1) that is expressed in the embryonic precursor of the placodes, the pre-placodal ectoderm (PPE). This gene is highly expressed in all neurogenic cranial placodes and lateral line primordia from neurula to tadpole stages. We used markers of the PPE to show that low concentrations of neural inducers (e.g., Noggin, Chordin) are necessary to induce the PPE and that posteriorizing signals (e.g., Wnt, FGF) repress Six1 so it is expressed only in the head. Over-expression of Six1 expands the PPE at the expense of neural crest and epidermis, whereas knock-down of Six1 protein results in reduction of the PPE domain and expansion of the neural plate, neural crest and epidermis. Using activator and repressor constructs of Six1 or co-expression of wild-type six1 with activating (eya1) or repressing (groucho) co-factors, we demonstrated that Six1 inhibits neural crest and epidermis genes via transcriptional repression and enhances PPE genes via transcriptional activation. Ectopic expression of neural plate, neural crest and epidermal genes in the PPE demonstrates that these factors mutually influence each other to establish the appropriate boundaries between these ectodermal domains. We are continuing to examine the upstream regulators of Six1 expression and the down-stream target genes.
In collaboration with Gerhart Schlosser and Mike Klymkowski, we studied whether Six1 plays a role in controlling cell proliferation and differentiation during later placode development. Six1 is expressed in both superficial and sensorial layers of the neurogenic placodes beginning at mid-gastrula stages, but the neuronal derivatives turn off Six1 expression as they migrate away from the placodal region. We showed that both Six1 and Eya1 are required for neural differentiation in all neurogenic placodes. At high levels of expression, Six1 and Eya1 expand the expression of SoxB1 genes, maintain cells in proliferative state and block expression of neuronal differentiation genes. At lower levels, they promote neuronal differentiation.
The transcriptional activity of Six proteins can be modified by co-factor proteins, the best characterized being Eya and Groucho proteins. We searched the Xenopus genome for orthologues of Drosophila co-factor proteins that interact with the fly six-related factor SO. We identified 33 Xenopus genes with high sequence identity to 20 fly proteins and demonstrate that a large number of these are expressed in craniofacial tissues that express Six1. Currently we are characterizing the developmental functions of these cofactors.
-
Related Articles
-
- Neal, S.J., Rajasekaran, A., Jusic, N., Taylor, L., Read, M., Alfandari, D., Pignoni, F. and S.A. Moody (2023) Using Xenopus to discover new genes involved in congenital hearing loss syndromes. J. Experimental Zoology, Part B. doi.org/10.1002/jez.b.23222.
- Jourdeuil, K., K.M. Neilson, H. Cousin, A.L.P. Tavares, H.D. Majumdar, D. Alfandari and S.A. Moody (2023) Zmym4 is required for early cranial gene expression and craniofacial cartilage formation. Frontiers in Cell and Developmental Biology 11: 1274788. doi: 10.3389/fcell.2023.1274788.
- Baxi, A.B., P. Nemes and S.A. Moody (2023) Time-resolved quantitative proteomic analysis of the developing Xenopus otic vesicle reveals putative congenital hearing loss candidates. iScience 26. doi:10.1016/j.isci.2023.107665.
- Klein, S.L., A.L.P. Tavares, M. Peterson, C.H. Sullivan and S.A. Moody (2022) Repressive interactions between transcription factors separate different ectodermal domains. Frontiers in Cell and Developmental Biology 10: 786052. doi: 10.3389/fcell.2022.786052. PMID: 35198557. PMCID: PMC8859430
- Keer, S., H. Cousin, K. Jourdeuil, K.M. Neilson, A.L.P. Tavares, D. Alfandari and S.A. Moody (2022) Mcrs1 is required for branchial arch and cranial cartilage development. Developmental Biology 489: 62-75. doi: 10.1016/j.ydbio.2022.06.002. PMID: 35697116. PMC10426812
- Coppenrath, K., A.L.P. Tavares, N.-I. Shaidani, M. Wlizla, S.A. Moody and M. Horb (2021) Generation of a new six1-null line in Xenopus tropicalis for study of development and congenital disease. genesis, The Journal of Genetics and Development doi: 10.1002/DVG.23453. PMID: 34664392
- Tavares, A.L.P., K. Jourdeuil, K.M. Neilson, H.D. Majumdar and S.A. Moody (2021) Sobp modulates Six1 transcriptional activation and is required during craniofacial development. Development 148: dev199684 doi: 10.1242/dev.199684. PMID: 34414417.
- Mehdizadeh, T., H.D. Majumdar, S. Ahsan, A.L.P. Tavares and S.A. Moody (2021) Mutations in SIX1 associated with Branchio-oto-renal Syndrome (BOR) differentially affect otic expression of putative target genes. J. Developmental Biology 9: 25. doi: 10.3390/jdb9030025. PMID: 34208995.
- Neilson, K.M., S. Keer, N. Bousquet, H.D. Majumdar, K.L. Kenyon, D. Alfandari and S.A. Moody (2020) Mcrs1 interacts with Six1 to influence early craniofacial and otic development Developmental Biology 467: 39-50. doi: 10.1016/j.ydbio.2020.08.013. PMID: 32891623.
- Shah, A.M., P. Krohn, A.P. Baxi, A.L.P. Tavares, C.H. Sullivan, Y.R. Chillakuru H.D. Majumdar, K.M. Neilson and S.A. Moody (2020) Six1 proteins with human Branchio-oto-renal mutations differentially affect cranial gene expression and otic development. Disease Models & Mechanisms 13: dmm043489. doi: 10.1242/dmm.043489. PMID: 31980437.
- Sullivan, C.H., H.D. Majumdar, K.M. Neilson and S.A. Moody (2019) Six1 and Irx1 have reciprocal interactions during cranial placode and otic vesicle formation. Developmental Biology 446: 68-79. doi: 10.1016/j.ydbio.2018.12.003. PMID: 30529252.
- Neilson, K.M., Abbruzzesse, G., Kenyon, K.L., Bartolo, V., Krohn, P., Alfandari, D., and S.A. Moody (2017) Pa2G4 is a novel Six1 co-factor that is required for neural crest and otic development. Developmental Biology 421: 171-182. PMID: 27940157
- Moody, S.A. and A.-S. LaMantia (2015) “Transcriptional regulation of cranial sensory placode development” Current Topics in Developmental Biology 111: 301-350. PMID: 25662264. Link
- Moody, S.A., Neilson, K.M., Kenyon, K.L., Alfandari, D., and F. Pignoni (2015) “Using Xenopus to discover new genes involved in Branchiootorenal spectrum disorders” Comparative Biochemistry and Physiology. Part C. Toxicol. Pharmacol. 178: 16-24. PMID: 26117063. Link
- Yan, B., Neilson, K.M., Ranganathan, R., Streit, A., and S.A. Moody (2015) Microarray identification of novel genes downstream of Six1, a critical factor in cranial placode, somite and kidney development. Developmental Dynamics 244: 181-210. PMID: 25403746. Link
- Saint-Jeannet, J.-P. and S.A. Moody (2014) “Establishing the pre-placodal region and breaking it into placodes with distinct identities” Developmental Biology 389: 13-27. PMCID: PMC3985045. Link
- Moody, S.A. and J.-P. Saint-Jeannet (2014) “Determination of pre-placodal ectoderm and sensory placodes” In: Principles of Developmental Genetics. Elsevier, NY. Second edition, pp 331-356.
- Gee, S.T., S.L. Milgram, K.L. Kramer, F.L. Conlon and S.A. Moody. (2011) Yes-associated protein 65 (YAP) expands neural progenitors and regulates pax3 expression in the neural plate border zone. PLoS One 10.1371/journal.pone.0020309. Link
- Neilson, K.M., F. Pignoni, B. Yan and S.A. Moody. (2010) Developmental expression patterns of candidate co-factors for vertebrate Six family transcription factors. Developmental Dynamics 239: 3446-3466. Link
- Schlosser, G., T. Awtry, S.A. Brugmann, ED. Jensen, K.M. Neilson, G. Ruan, A. Stammler, D. Voelker, B.Yan, C. Zhang, M.W. Klymkowsky, and S.A. Moody. (2008). Eya1 and Six1 promote neurogenesis in the cranial placodes in a SoxB1?dependent fashion. Developmental Biology 320:199?214.Link
- Moody, S.A. (2007). Determination of pre-placodal ectoderm and sensory placodes. In: Principles in Developmental Genetics. Elsevier, NY, pp. 590-614. Link
- Brugmann, S.A. and S.A. Moody (2005). Induction and specification of the vertebrate ectodermal placodes: precursors of the cranial sensory organs. Biol. Cell 97: 303-319. Link
- Brugmann, S.A., P.D. Pandur, K.L. Kenyon, F. Pignoni and S.A. Moody (2004). Six1 promotes a placodal fate within the lateral neurogenic ectoderm by functioning as both a transcriptional activator and repressor. Development 131: 5871-5881. Link
- Pandur, P., and S.A. Moody (2000). Xenopus six1 gene is expressed in neurogenic cranial placodes and maintained in differentiating lateral lines. Mechanisms of Development 96: 253-257. Link
Cranial Nerve Development and Dysphagia
Using the LgDel mouse model of 22q11 deletion (Di George) syndrome, a team of investigators, including Dr. Moody, are investigating the developmental causes of feeding and swallowing difficulties (called pediatric dysphagia) experienced by these patients.
-
Related Articles
-
- Karpinski, B.A., T.M. Maynard, C.A. Bryan, G. Yitsege, A. Horvath, N.H. Lee, S.A. Moody and A.-S. LaMantia (2022) Selective disruption of trigeminal sensory neurogenesis and differentiation in a mouse model of 22q11.2 Deletion Syndrome. Disease Models & Mechanisms 15: dmm 047357. doi: 10.1242/dmm.047357. PMID: 33722956.
- Maynard, T.M., A. Horvath, J. Bernout, B. Karpinski, A.L.P. Tavares Q. Zheng, L. Spurr, J. Olender, S.A. Moody, C.M. Fraser, A.-S. LaMantia and N.H. Lee (2020) Transcriptional dysregulation in developing trigeminal sensory neurons in the LgDel mouse model of DiGeorge 22q11.2 deletion syndrome. Human Molecular Genetics 29: 1002-1017. doi: 10.1093/hmg/ddaa024. PMID: 32047912.
- Motahari, Z., A. Popratiloff, E.M. Paronett, T.M. Maynard, S.A. Moody and A.-S. LaMantia (2020) Aberrant early growth of individual trigeminal sensory and motor axons in multiple mouse genetic models of 22q11.2 deletion syndrome. Human Molecular Genetics 29: 3081-3093. doi: 10.1093/hmg/ddaa199. PMID: 32901287.
- Motahari, Z., S.A. Moody, T.M. Maynard and A.-S. LaMantia (2019) In the Line-up: Deleted genes associated with DiGeorge/22q11.2 Deletion Syndrome: Are they all suspects? J. Neurodevel. Disorders 11:7 doi: 10.1186/s11689-019-9267-z. PMID:31174463.
- Karpinski, B.A., Bryan, C., Paronett, E., Baker, J., Fernandez, A., Horvath, A., Maynard, T.M., Moody, S.A., and A.-S. LaMantia (2016) A cellular and molecular mosaic establishes growth and differentiation states for cranial sensory neurons. Developmental Biology 415: 228-241. PMID: 26988119.
- LaMantia, A.-S., Moody, S.A., Maynard, T., Karpinski, B.A., Zohn, I., Mendelowitz, D., Lee, N.H. and A. Popratiloff (2015) “Hard to swallow: Developmental biological insights into pediatric dysphagia” Developmental Biology, 409: 329-342. PMID: 26554723. Link
- Karpinski, B.A., Maynard, T.M., Fralish, M.S., Nuwayhid, S., Zohn, I., Moody, S.A. and A.S. LaMantia (2014) Dysphagia and disrupted cranial nerve development in a mouse model of DiGeorge/22q11 Deletion Syndrome. Disease Models and Mechanisms 7:245-257. PMCID: PMC3917245. Link
Specification of Retinal Stem Cells and Amacrine Cell Phenotypes
The ability of embryonic cells to give rise to the retina is influenced by both maternal molecules and cell-cell interactions throughout development. We demonstrated that maternal asymmetries that set up the three embryonic germ layers (ectoderm, mesoderm, endoderm) influence whether an embryonic cell can give rise to retina. Vegetally localized factors that promote endo-mesoderm formation also antagonize neural and retinal fates involving the Derriere signaling pathway. Those blastomeres that do give rise to the retina are selected from the pool of competent blastomeres by residing in a region of the embryo where BMP signaling is highly repressed.
-
Related Articles
-
- Yan, B. and S.A. Moody (2007). The competence of Xenopus blastomeres to produce neural and retinal progeny is repressed by two endo-mesoderm promoting pathways. Developmental Biology 305:103-119. Link
- Zaghloul, N.A., Yan, B. and S.A. Moody (2005). “Step-wise specification of retinal stem cells during normal embryogenesis” Biol. Cell 97:321-337. Link
- Moore, K.B. and S.A. Moody (1999). Animal-vegetal asymmetries influence the earliest steps in retinal fate commitment in Xenopus. Developmental Biology 212:25-41. Link
During gastrulation retinal stem cells are selected from the pool of retina-competent precursors to form the definitive eye fields. Cells in the eye fields express several transcription factors that specify their retinal fate. We show that eye-specific transcription factors and ephrinB1-FGF signaling influence which embryonic cells are able to move into the eye field. We also showed that two of these factors, Rx1 and Pax6, differentially influence whether a cell maintains an immature retinal stem cell state or becomes a more restricted retinal progenitor cell.
-
Related Articles
-
- Zaghloul, N.A. and S.A. Moody (2007). Alterations of rx1 and pax6 expression levels at neural plate stages differentially affect the production of retinal cell types and maintenance of retinal stem cell qualities. Developmental Biology 306: 222-240. Link
- Lee, H.-S., Y.-S. Bong, K B. Moore, K. Soria, S.A. Moody and I.O. Daar (2006). Dishevelled mediates ephrinB1 signaling in the eye field via the planar cell polarity pathway. Nature Cell Biology 8: 55-63. Link
- Moore, K.B., K. Mood, I.O. Daar and S.A. Moody (2004). Morphogenetic movements underlying eye field formation require interactions between the FGF and ephrinB1 signaling pathways. Developmental Cell 6: 55-67.Link
- Kenyon, K.L., N. Zaghloul and S.A. Moody (2001). Transcription factors of the anterior neural plate alter cell movements of epidermal progenitors to specify a retinal fate. Developmental Biology 240: 77-91. Link
Using cell lineage analyses, neurotransmitter-specific immunocytochemistry, and confocal microscopy, we determined the cell lineage origin of different neurotransmitter subtypes of amacrine neurons. Using single cell transplantation and gene mis-expression techniques, we demonstrated some of the interactions and transcription factors necessary to produce these specific phenotypes.
-
Related Articles
-
- Moody, S.A. (2012) “Targeted microinjection of synthetic mRNAs to alter retina gene expression in Xenopus embryos.” In: Methods in Molecular Biology: Retinal Development 884: 91-111. Link
- Moody, S.A. (2012) “Testing retina fate commitment in Xenopus by blastomere deletion, transplantation and explant culture.” In: Methods in Molecular Biology: Retinal Development 884: 115-127. Link
- Zaghloul, N.A. and S.A. Moody (2007). Changes in Rx1 and Pax6 activity at eye field stages differentially alter the production of amacrine neurotransmitter subtypes in Xenopus. Molecular Vision 13: 86-95. Link
- Moody, S.A., I. Chow and S. Huang (2000). Intrinsic bias and lineage restriction in the phenotype determination of dopamine and neuropeptide Y amacrine cells. J. Neuroscience 20: 3244-3253. Link
- Huang, S. and S.A. Moody (1998). Dual expression of GABA or serotonin and dopamine in Xenopus amacrine cells is transient and may be regulated by laminar cues. Visual Neuroscience 15: 969-977. Link
- Huang, S. and S.A. Moody (1997). Three types of serotonin-containing amacrine cells in the tadpole retina have distinct clonal origins. J. Comparative Neurology 387: 42-52. Link
- Huang, S. and S.A. Moody (1995). Asymmetrical blastomere origin and spatial domains of dopamine and Neuropeptide Y amacrine cells in Xenopus tadpole retina. J. Comparative Neurology 360: 2-13. Link
- Huang, S. and S.A. Moody (1993). The retinal fate of Xenopus cleavage stage progenitors is dependent upon blastomere position and competence: Studies of normal and regulated clones. J. Neuroscience 13: 3193-3210. Link
The Upstream Regulatory Regions of a Neuron-Specific β-tubulin Gene
By immunocytochemistry we and collaborators showed that the Class III β-tubulin gene is expressed only in neurons beginning at the time that they go through their terminal cell division. Therefore, this gene is likely to be regulated by proteins that establish neuronal cell fate. We cloned the upstream sequences of this gene in rat and analyzed in silico which regions are likely to be involved in neuron-specific expression.
-
Related Articles
-
- Dennis, K., M. Uittenbogaard, A. Chiaramello, and S.A. Moody (2002). The promoter region of the rat neuron-specific Class III beta-tubulin gene contains several proximal elements that may regulate neuron specific expression. Gene 294: 269-277. Link
- Moody, S.A., V. Miller, A. Spanos and A. Franfurter (1996). Developmental expression of neuron-specific beta-tubulin in frog (Xenopus laevis); a marker for growing axons during the embryonic period. J. Comparative Neurology 364: 219-230. Link
- Moody, S.A., M.S. Quigg and A Frankfurter (1989). Development of the peripheral trigeminal system in the chick revealed by an isotype-specific anti-beta-tubulin monoclonal antibody. J. Comparative Neurology 279: 567-580. Link
To acquire the frog neuron-specific anti-beta-tubulin monoclonal antibody, contact af6a [at] virginia [dot] edu (Dr. Anthony Frankfurter) at the University of Virginia.
To acquire clones of the rat neuron-specific Class III beta-tubulin gene upstream sequences, contact samoody [at] gwu [dot] edu (Dr. Moody).
Fate Maps in Xenopus laevis
The first use of intracellular lineage tracers to make fate maps in Xenopus was published by Marcus Jacobson and Giro Hirose (1978, Science 202: 637-639).
The following articles contain the fate maps of Xenopus laevis blastomeres and specific neuronal phenotypes.
-
Related Articles
-
- Klein, S. L. and S.A. Moody (2016) “When family history matters: the importance of lineage analyses and fate maps for explaining animal development” Current Topics in Developmental Biology 117: 93-112. (invited for the 50th anniversary volume). PMID: 26969974. Link
- Lee, H.-S., Sokol, S.Y., Moody, S.A, and I. O. Daar (2012) “Using 32-cell stage Xenopus embryos to probe PCP signaling.” In: Methods in Molecular Biology: PCP Methods and Protocols 839: 91-104. Link
- Moody, S.A. (1999) "Cell Lineage Analysis in Xenopus Embryos" In: Methods in Molecular Biology: Developmental Biology Protocols, (ed., R.S. Tuan and C.W. Lo) Humana Press, v 135, pp 1-17.
- Moody, S.A. (1999) Testing the Cell Fate Commitment of Single Blastomeres in Xenopus laevis. In: Advances in Molecular Biology, (ed., J. Richter), Oxford University Press, pp. 355-381.
- Bauer, D.V., S. Huang and S.A. Moody (1994). The cleavage stage origin of Spemann's Organizer: Analysis of the movements of blastomere clones before and during gastrulation in Xenopus. Development 120: 1179-1189. Link
- Huang, S. and S.A. Moody (1993). The retinal fate of Xenopus cleavage stage progenitors is dependent upon blastomere position and competence: Studies of normal and regulated clones. J. Neuroscience 13: 3193-3210. Link
- Huang, S. and S.A. Moody (1992). Does lineage determine the dopamine phenotype in the tadpole hypothalamus: A quantitative analysis. J. Neuroscience 12: 1351-1362. Link
- Moody, S.A. and M.J. Kline (1990). Segregation of fate during cleavage of frog (Xenopus laevis) blastomeres. Anatomy & Embryology 182: 347-362. Link
- Moody, S.A. (1989). Quantitative lineage analysis of the origin of frog primary motor and sensory neurons from cleavage stage blastomeres. J. Neuroscience 9: 2919-2930. Link
- Hirose, G. and M. Jacobson (1979). Clonal organization of the central nervous system of the frog. I. Clones stemming from individual blastomeres of the 16-cell and earlier stages. Developmental Biology 71: 191-202. Link
- Jacobson, M. and G. Hirose (1978). Origin of the retina from both sides of the embryonic brain: A contribution to the problem of crossing at the optic chiasma. Science 202: 637-639. Link
- Moody, S.A. (1987). Fates of the blastomeres of the 16-cell stage Xenopus embryo. Developmental Biology 119: 560-578. Link
- Jacobson, M. and S.A. Moody (1984). Quantitative lineage analysis of the frog's nervous system. I. Lineages of Rohon-Beard neurons and primary motoneurons. J. Neuroscience 4: 1361-1369. Link
- Jacobson, M. (1983). Clonal organization of the central nervous system of the frog. III. Clones stemming from individual blastomeres of the 128- , 256- , and 512-cell stages. J. Neuroscience 3:1019-1038. Link
- Jacobson, M. and G. Hirose (1981). Clonal organization of the central nervous system of the frog II. Clones stemming from individual blastomeres of the 32- and 64-cell stages. J. Neuroscience 1: 271-284. Link
Books
- Moody, S.A. (1998) “Cell Lineage and Fate Determination” Academic Press, NY. ISBN: 0-12-505255-3.
- Moody, S.A. (2007) “Principles of Developmental Genetics” 1st edition, Elsevier, NY. ISBN: 0-12-369548-1. Awarded a 5-star rating by Doody Enterprise’s Book Review Service.
- Moody, S.A. (2014) “Principles of Developmental Genetics” 2nd edition, Elsevier, NY. ISBN-13: 978-0124059450 ISBN-10: 0124059457. Selected as a Core Title in Clinical Genetics by Doody Enterprise’s Book Review Service.
- Hall, B.K. and S.A. Moody (2018) “Translating Genotypes into Phenotypes – Past, Present and Future”. CRC Press “Cells in Evolutionary Biology” book series, Volume 1. https://www.crcpress.com/Cells-in-Evolutionary-Biology-Translating-Genotypes-into-Phenotypes---Past/Hall-Moody/p/book/9…. ISBN 978-1498787864
- Fainsod, A. and S.A. Moody (2022) “Xenopus, from basic biology to disease models in the genomic era” CRC Press. ISBNs: 978-0367505271 (hardback), 978-0367505349 (paperback), 978-1003050230 eBook.
- https://www.routledge.com/Xenopus-From-Basic-Biology-to-Disease-Models-in-the-Genomic-Era/Fainsod-Moody/p/book/97803675…
-
- Publications
-
2023-2021
Shukla, D., B.M. Gural, E.S. Cauley, L.E. Roberts B. F. Karas, L. Cavallo, L., Turkalj, S.A. Moody, L.E. Swan and M.C. Manzini. (2023) Duplicated zebrafish (Danio rerio) inositol Phosphatase inpp5ka and inpp5kb diverged in expression pattern and function. Development, Genes & Evolution 233: 25-34. PMID: 37184573. PMCIDF: PMC10239392.
Marchak, A., K.M. Neilson, H. D. Majumdar, S.L. Klein and S.A. Moody (2023) The sulfotransferase XB5850668.L is required to apportion embryonic ectodermal domains. Developmental Dynamics doi: 10.1002/dvdy.648. PMID: 37597164
Baxi, A.B., P. Nemes and S.A. Moody (2023) Time-resolved quantitative proteomic analysis of the developing Xenopus otic vesicle reveals putative congenital hearing loss candidates. iScience 26. doi:10.1016/j.isci.2023.107665.
Jourdeuil, K., K.M. Neilson, H. Cousin, A.L.P. Tavares, H.D. Majumdar, D. Alfandari and S.A. Moody (2023) Zmym4 is required for early cranial gene expression and craniofacial cartilage formation. Frontiers in Cell Developmental Biology 11: 1274788. doi: 10.3389/fcell.2023.1274788.
Keer, S., K.M. Neilson, H.D. Majumdar, S.L. Klein, and S.A. Moody (2023) Bop1 is required to establish precursor domains of craniofacial tissues. genesis, The Journal of Genetics and Development (under revision).
Neal, S.J., Rajasekaran, A., Jusic, N., Taylor, L., Read, M., Alfandari, D., Pignoni, F. and S.A. Moody (2023) Using Xenopus to discover new genes involved in congenital hearing loss syndromes. J. Experimental Zoology, Part B. doi.org/10.1002/jez.b.23222.
Tavares, A.L.P. and S.A. Moody (2022) "Advances in understanding the pathogenesis of craniofacial birth defects" J. Developmental Biology 10:27. doi: 10.3390/jdb10030027.
Keer, S., H. Cousin, K. Jourdeuil, K.M. Neilson, A.L.P. Tavares, D. Alfandari and S.A. Moody (2022) Mcrs1 is required for branchial arch and cranial cartilage development. Developmental Biology 489:62-75. doi: 10.1016/j.ydbio.2022.06.002. PMID: 35697116. PMC10426812
Zahn, N., C. James-Zorn, D.S. Adams, V.G. Ponferrada, D.R. Buchholz, M. Horb, S. A. Moody, P. Vize and A.M. Zorn (2022) Normal Table of Xenopus development: a new graphical resource. Development149: dev200356. PMID: 35833709. PMCIPD: PMC9445888.
Leibovich, A., Moody, S.A., Klein, S.L. and A. Fainsod (2022) Xenopus: a Model to Study Natural Genetic Variation and its Disease Implications. In: “Xenopus, from basic biology to disease models in the genomic era” CRC Press. Boca Raton. pp 313-324.
Karpinski, B.A., Maynard, T.M., Bryan, C.A., Yitsege, G., Horvath, A., Lee, N.H., Moody, S.A., and A.S. LaMantia (2022) Selective disruption of trigeminal sensory neurogenesis and differentiation in a mouse model of 22q11.2 Deletion Syndrome. Disease Models & Mechanisms 15: dmm 047357. doi: 10.1242/dmm.047357. PMID: 33722956.
Klein, S.L., Tavares, A.L.P., Peterson, M., Sullivan, C.H., and S.A. Moody (2022) Repressive interactions between transcription factors separate different ectodermal domains. Frontiers in Cell and Developmental Biology 10: 786052. doi: 10.3389/fcell.2022.786052
Gur, M., Edri, T., Moody, S.A. and A. Fainsod (2022) Retinoic acid is required for normal morphogenetic movements during gastrulation. Frontiers in Cell and Developmental Biology 10: 857230. doi: 10.3389/fcell.2022.857230.
Onjiko, R.M., Nemes, P., and S.A. Moody (2021) Altering metabolite distribution at Xenopus cleavage stages affects left-right gene expression asymmetries. genesis, The Journal of Genetics and Development, 59: e23418. doi: 10.1002/dvg.23418. PMID: 33826226.
Brugmann, S., Clouthier, D.E., Saint-Jeannet, J.P., Taneyhill, L.A., and Moody, S.A. (2021) The Society for Craniofacial Genetics and Developmental Biology 43rd Annual Meeting. Amer. J. Med. Genetics, Part A. 185: 1932-1939. doi: 10.1002/ajmg.a.62150.
Moody, S.A. (2021) Xenopus explants and transplants. Cold Spring Harbor Protocols, doi 10:1101/pdb.top097337.
Mehdizadeh, T., Majumdar, H.D., Ahsan, S., Tavares, A.L.P. and S.A. Moody (2021) Mutations in SIX1 associated with Branchio-oto-renal Syndrome (BOR) differentially affect otic expression of putative target genes. J. Developmental Biology 9: 25. doi: 10.3390/jdb9030025. PMID: 34208995.
Tavares, A.L.P., Jourdeuil, K., Neilson, K.M., Majumdar, H.D. and S.A. Moody (2021) Sobp modulates Six1 transcriptional activation and is required during craniofacial development. Development 148: dev199684 doi: 10.1242/dev.199684 PMID: 34414417.
Coppenrath, K., Tavares, A.L.P., Shaidani, N-I., Wlizla, M., Moody, S.A., and M. Horb (2021) Generation of a new six1-null line in Xenopus tropicalis for study of development and congenital disease. genesis, The Journal of Genetics and Development doi: 10.1002/DVG.23453. PMID: 34664392
2020 - 2017
Maynard, T.M., Zohn, I.E., Moody, S.A. and LaMantia, A.S. (2020) Suckling, Feeding and Swallowing: Behaviors, Circuits and Targets for Neurodevelopmental Pathology. Ann. Rev. Neuroscience 43: 315-336. PMID: 32101484.
Eisenhoffer, G.T., Clouthier, D., Cox, T., Saint-Jeannet, J.P., Taneyhill, L.A., Trainor, P.A. and Moody, S.A. (2020) The Society for Craniofacial Genetics and Developmental Biology 42nd Annual Meeting. Amer. J. Med. Genetics, Part A. 187: 1555-1561. doi 10.1002/ajmg.a.61602
Shah, A.M., Krohn, P., Baxi, A.P., Tavares, A.L.P., Sullivan, C.H., Chillakuru Y.R., Majumdar, H.D., Neilson, K.M., and S.A. Moody (2020) Six1 proteins with human Branchio-oto-renal mutations differentially affect cranial gene expression and otic development. Disease Models & Mechanisms 13: dmm043489. doi: 10.1242/dmm.043489. PMID: 31980437.
Maynard, T.M., Horvath A., Bernout, J., Karpinski, B., Tavares A.L.P., Zheng, Q., Spurr, L., Olender, J., Moody, S.A., Fraser, C.M., A.S. LaMantia, and N.H. Lee (2020) Transcriptional dysregulation in developing trigeminal sensory neurons in the LgDel mouse model of DiGeorge 22q11.2 deletion syndrome. Human Molecular Genetics 29: 1002-1017. doi: 10.1093/hmg/ddaa024. PMID: 32047912.
Leibovich, A. Edri, T., Klein, S.L., Moody, S.A. and A. Fainsod (2020) Natural variation among embryos leads to corresponding scaling in gene expression. Developmental Biology 462: 165-179. doi: 10.1016/j.ydbio.2020.03.014. PMID: 32259520.
Moody, S.A. and S.L. Klein (2019) Deferred-use molecules and decision-making in development. In: Deferring development: Setting aside cells for future use in development and evolution. C. Bishop and B. K. Hall Eds., CRC Press, pp 29-52.
Moody, S.A. (2019) Cleavage blastomere deletion and transplantation. Cold Spring Harbor Protocols doi: 10.1101/pdb.prot097311. PMID: 29769398.
Moody, S.A. (2019) Cleavage blastomere explant culture. Cold Spring Harbor Protocols doi: 10.1101/pdb.prot097303. PMID: 29769392.
Moody, S.A. (2019) Analysis of Cell Fate Commitment in Xenopus Embryos. Cold Spring Harbor Protocols doi: 10.1101/pdb.top097246. PMID: 29769394.
Motahari, Z., Moody, S.A., Maynard, T.M., LaMantia, A.S. (2019) In the Line-up: Deleted genes associated with DiGeorge/22q11.2 Deletion Syndrome: Are they all suspects? J. Neurodevel. Disorders 11:7 doi: 10.1186/s11689-019-9267-z. PMID:31174463.
Sullivan, C.H., Majumdar, H.D., Neilson, K.M., and S.A. Moody (2019) Six1 and Irx1 have reciprocal interactions during cranial placode and otic vesicle formation. Developmental Biology 446: 68-79. doi: 10.1016/j.ydbio.2018.12.003. PMID: 30529252.
Lombard-Banek, C., Moody, S.A., Manzini, M.C., and P. Nemes (2019) Microsampling capillary electrophoresis mass spectrometry enables single-cell proteomics in complex tissues: Developing cell clones in live Xenopus laevis and zebrafish embryos. Analytical Chemistry 91: 4797-4805. doi: 10.1021/acs.analchem.9b00345. PMID: 30827088.
Taneyhill, L.A., Moody, S.A., Cox, T., Klein, O., Marcucio, R., Schneider, R. and Trainor, P.A. (2019) The Society for Craniofacial Genetics and Developmental Biology 41st Annual Meeting. Amer. J. Med. Genetics, Part A. 179: 864-869. doi: 10.1002/ajmg.a.61090. PMID: 30793834
Baxi, A., Lombard-Banek, C., Moody, S.A., and P. Nemes (2018) Proteomic characterization of the neural ectoderm fated cell clones in the Xenopus laevis embryo by high-resolution mass spectrometry. ACS Chemical Neuroscience 9: 2064-2073. doi: 10.1021/acschemneuro.7b00525. PMID: 29578674.
Moody, S.A. (2018) Lineage tracing and fate mapping. Cold Spring Harbor Protocols doi: 10.1101/pdb.prot097253. PMID: 29769388.
Moody, S.A. (2018) Microinjection of mRNAs and oligonucleotides. Cold Spring Harbor Protocols doi: 10.1101/pdb.prot097261. PMID: 29769401.
Gammill, L., Cox, T., Moody, S., Taneyhill, L., Trainor, P., and Marcucio, R. (2018) The Society for Craniofacial Genetics and Developmental Biology 40th annual meeting. Amer. J. Med. Genet. Part A, 176: 1270-1273. doi: 10.1002/ajmg.a.38653. PMID: 29681098.
Moody, S.A. (2018) Case study 14: Illmensee and Mahowald, 1974, Pole plasm. In: Ahead of the Curve: Hidden Breakthroughs in the Biosciences. Volume 2. D.S. Adams and M. Levin Eds., Bristol, UK, Institute of Physics Publishing, pp.4-1 to 4-3.
Sater, A.K. and S.A. Moody (2017) Using Xenopus to understand human disease and developmental disorders. genesis, The Journal of Genetics and Development 55. doi: 10.1002/dvg.22997. PMID: 28095616.
Neilson, K.M., Abbruzzesse, G., Kenyon, K.L., Bartolo, V., Krohn, P., Alfandari, D., and S.A. Moody (2017) Pa2G4 is a novel Six1 co-factor that is required for neural crest and otic development. Developmental Biology 421: 171-182. PMID: 27940157
Onjiko, R., Plotnick, D.O., Moody, S.A., and P. Nemes (2017) Metabolic comparison of dorsal versus ventral cells directly in the live 8-cell frog embryo by microprobe single-cell CE-ESI-MS. Analytical Methods 9: 4964-4970. PMID: 29062391.
Onjiko, R.M., Potero, E.P., Moody, S.A. and P. Nemes (2017) Microprobe capillary electrophoresis mass spectrometry for single-cell metabolomics in live frog (Xenopus laevis) embryos. J. Vis. Experiments 130: e56956, doi:10.3791/56956. PMID:29286491.
Sherman, J.H., Karpinski, B., Fralish, M., Cappuzzo, J., Dhindsa, D., Thal, A. Moody, S.A., LaMantia, A.S., and T.M. Maynard (2017) Foxd4 is essential for establishing neural cell fate and for neuronal differentiation. genesis, The Journal of Genetics and Development 55: ePub. doi: 10.1002/dvg.23031. PMID: 28316121 (Cover article)
Onjiko, R., Portero, E., Moody, S.A., and P. Nemes (2017) In situ microprobe capillary electrophoresis mass spectrometry: metabolic reorganization in single differentiating cells in the live vertebrate (X. laevis) embryo. Analytical Chemistry 89: 7069-7076. PMID: 28434226 (Cover article)
Marchak, A., Grant, P., Neilson, K.M., Majumdar, H.D., Yaklichkin, S., Johnson, D., and S.A. Moody (2017). Wbp2nl has a developmental role in establishing neural and non-neural ectodermal fates. Developmental Biology 429: 213-224. PMID: 28663133.
2016 - 2013
Klein, S. L. and S.A. Moody (2016) “When family history matters: the importance of lineage analyses and fate maps for explaining animal development” Current Topics in Developmental Biology 117: 93-112. (invited for the 50th anniversary volume). PMID: 26969974. Link
Lombard-Banek, C., Moody, S.A., and P. Nemes (2016) Single-cell mass spectrometry for discovery proteomics: quantifying translational cell heterogeneity in the 16-cell frog (Xenopus) embryo. Angewandte Chemie International Edition 55: 2454-2458. PMID: 26756663 Link
Karpinski, B.A., Bryan, C., Paronett, E., Baker, J., Fernandez, A., Horvath, A., Maynard, T.M., Moody, S.A., and A.-S. LaMantia (2016) A cellular and molecular mosaic establishes growth and differentiation states for cranial sensory neurons. Developmental Biology 415: 228-241. PMID: 26988119 Link
Onjiko, R., Morris, S., Moody, S.A. and P. Nemes (2016) Single-cell mass spectrometry with multi-solvent extraction identified metabolic differences between left and right blastomeres in the 8-cell frog (Xenopus) embryo. Analyst 141: 3648-3656. PMID: 27004603 Link
Gaur, S., Mandelbaum, M., Herold, M., Majumdar, H.D., Neilson, K.M., Maynard, T.M., Mood, K., Daar, I.O. and S.A. Moody (2016) Neural transcription factors bias cleavage stage blastomeres to give rise to neural ectoderm. genesis, The Journal of Genetics and Development 54: 334-349. PMID: 27092474 Link
Lombard-Banek, C., Reddi, S., Moody, S.A., and P. Nemes (2016) Label-free quantification of proteins in single embryonic cells by capillary electrophoresis high-resolution mass spectrometry. Molec. Cell. Proteomics Jun 17. pii: mcp.M115.057760. [Epub ahead of print]. PMID: 27317400 Link
Klein, S. L. and S.A. Moody (2016) “When family history matters: the importance of lineage analyses and fate maps for explaining animal development” Current Topics in Developmental Biology 117: 93-112. (invited for the 50th anniversary volume). PMID: 26969974. Link
Lombard-Banek, C., Reddi, S., Moody, S.A., and P. Nemes (2016) Label-free quantification of proteins in single embryonic cells by capillary electrophoresis high-resolution mass spectrometry. Molec. Cell. Proteomics Jun 17. pii: mcp.M115.057760. [Epub ahead of print]. PMID: 27317400 Link
Gaur, S., Mandelbaum, M., Herold, M., Majumdar, H.D., Neilson, K.M., Maynard, T.M., Mood, K., Daar, I.O. and S.A. Moody (2016) Neural transcription factors bias cleavage stage blastomeres to give rise to neural ectoderm. genesis, The Journal of Genetics and Development 54: 334-349. PMID: 27092474 Link
Karpinski, B.A., Bryan, C., Paronett, E., Baker, J., Fernandez, A., Horvath, A., Maynard, T.M., Moody, S.A., and A.-S. LaMantia (2016) A cellular and molecular mosaic establishes growth and differentiation states for cranial sensory neurons. Developmental Biology 415: 228-241. PMID: 26988119 Link
Onjiko, R., Morris, S., Moody, S.A. and P. Nemes (2016) Single-cell mass spectrometry with multi-solvent extraction identified metabolic differences between left and right blastomeres in the 8-cell frog (Xenopus) embryo. Analyst 141: 3648-3656. PMID: 27004603 Link
Lombard-Banek, C., Moody, S.A., and P. Nemes (2016) Single-cell mass spectrometry for discovery proteomics: quantifying translational cell heterogeneity in the 16-cell frog (Xenopus) embryo. Angewandte Chemie International Edition 55: 2454-2458. PMID: 26756663 Link
Lombard-Banek, C., Moody, S.A. and P. Nemes (2016) “High-sensitivity mass spectrometry for probing gene translation in single embryonic cells in the early frog (Xenopus) embryo. Frontiers in Cell and Developmental Biology, 4:100. doi: 10.3389/fcell.2016.00100. PMID: 27761436.
LaMantia, A.-S., Moody, S.A., Maynard, T., Karpinski, B.A., Zohn, I., Mendelowitz, D., Lee, N.H. and A. Popratiloff (2015) “Hard to swallow: Developmental biological insights into pediatric dysphagia” Developmental Biology, 409: 329-342. PMID: 26554723. Link
Moody, S.A. and S.L. Klein (2015) “Cell lineage tracing for understanding embryonic development” Current Topics in Developmental Biology (invited for the 50th anniversary volume titled: "Essays on Developmental Biology-2016", in preparation).
Moody, S.A., Neilson, K.M., Kenyon, K.L., Alfandari, D., and F. Pignoni (2015) “Using Xenopus to discover new genes involved in Branchiootorenal spectrum disorders” Comparative Biochemistry and Physiology. Part C. (http://dx.doi.org/10.1016/j.cbpc.2015.06.007).
Moody, S.A. and A.-S. LaMantia (2015) “Transcriptional regulation of cranial sensory placode development” Current Topics in Developmental Biology 111: 301-350. PMID: 25662264. Link
Onjiko, R.M., Moody, S.A., and P. Nemes (2015) Single-cell mass spectrometry reveals small molecules that affect cell fates in the 16-cell embryo. Proceedings of the National Academy of Sciences (USA) 112: 6545-6550. PMID: 25941375. Highlighted in Science Signaling; Recommended in F1000Prime Link
Klein, S.L. and S.A. Moody (2015) Early neural ectodermal genes are activated by Siamois and Twin during blastula stages. genesis, The Journal of Genetics and Development 53: 308-320. PMID: 25892704 Link
Yan, B., Neilson, K.M., Ranganathan, R., Streit, A., and S.A. Moody (2015) Microarray identification of novel genes downstream of Six1, a critical factor in cranial placode, somite and kidney development. Developmental Dynamics 244: 181-210. PMID: 25403746 Link
Klein, S.L. and S.A. Moody (2015) Early neural ectodermal genes are activated by Siamois and Twin during blastula stages. genesis, The Journal of Genetics and Development 53: 308-320. PMID: 25892704 Link
Onjiko, R.M., Moody, S.A., and P. Nemes (2015) Single-cell mass spectrometry reveals small molecules that affect cell fates in the 16-cell embryo. Proceedings of the National Academy of Sciences (USA) 112: 6545-6550. PMID: 25941375. Highlighted in Science Signaling; Recommended in F1000Prime Link
Moody, S.A. and A.-S. LaMantia (2015) “Transcriptional regulation of cranial sensory placode development” Current Topics in Developmental Biology 111: 301-350. PMID: 25662264. Link
Moody, S.A., Neilson, K.M., Kenyon, K.L., Alfandari, D., and F. Pignoni (2015) “Using Xenopus to discover new genes involved in Branchiootorenal spectrum disorders” Comparative Biochemistry and Physiology. Part C. (http://dx.doi.org/10.1016/j.cbpc.2015.06.007).
Moody, S.A. and S.L. Klein (2015) “Cell lineage tracing for understanding embryonic development” Current Topics in Developmental Biology (invited for the 50th anniversary volume titled: "Essays on Developmental Biology-2016", in preparation).
LaMantia, A.-S., Moody, S.A., Maynard, T., Karpinski, B.A., Zohn, I., Mendelowitz, D., Lee, N.H. and A. Popratiloff (2015) “Hard to swallow: Developmental biological insights into pediatric dysphagia” Developmental Biology, 409: 329-342. PMID: 26554723. Link
Moody, S.A. and Saint-Jeannet, J.-P. (2014) “Determination of pre-placodal ectoderm and sensory placodes.” Developmental Biology (under review)
Saint-Jeannet, J.-P. and S.A. Moody (2014) “Establishing the pre-placodal region and breaking it into placodes with distinct identities” Developmental Biology 389: 13-27. PMCID: PMC3985045. Link
Lee, H.-K., Lee, H.-S. and S.A. Moody (2014) “Neural transcription factors: from embryos to neural stem cells” Molecules and Cells 37: 705-712. PMCID: PMC4213760. Link
Saint-Jeannet, J.-P. and S.A. Moody (2014) “Establishing the pre-placodal region and breaking it into placodes with distinct identities” Developmental Biology 389: 13-27. PMCID: PMC3985045. Link
Moody, S.A. and Saint-Jeannet, J.-P. (2014) “Determination of pre-placodal ectoderm and sensory placodes.” Developmental Biology (under review)
Grant, P.A., Herold, M.B., and Moody, S.A. (2013) “Blastomere explants to test for cell fate commitment during embryonic development.” Journal of Visualized Experiments (71)e4458, doi: 10.3791/4458 Link
Moody, S.A., Klein S.L., Karpinksi B.A., Maynard T.M. and LaMantia, A.-S. (2013) “On becoming neural: what the embryo can tell us about differentiating neural stem cells.” Amer. J. Stem Cells 2: 74-94. Link
Klein, S.L., Neilson, K.M., Orban, J. Yaklichkin, S., Hoffbauer, J., Mood, K., Daar, I.O. and Moody, S.A. (2013) “Conserved structural domains in FoxD4L1, a neural forkhead box transcription factor, are required to repress or activate target genes.” Plos One Link
Moody, S.A., Klein S.L., Karpinksi B.A., Maynard T.M. and LaMantia, A.-S. (2013) “On becoming neural: what the embryo can tell us about differentiating neural stem cells.” Amer. J. Stem Cells 2: 74-94. Link
Grant, P.A., Herold, M.B., and Moody, S.A. (2013) “Blastomere explants to test for cell fate commitment during embryonic development.” Journal of Visualized Experiments (71)e4458, doi: 10.3791/4458 Link
Klein, S.L., Neilson, K.M., Orban, J. Yaklichkin, S., Hoffbauer, J., Mood, K., Daar, I.O. and Moody, S.A. (2013) “Conserved structural domains in FoxD4L1, a neural forkhead box transcription factor, are required to repress or activate target genes.” Plos One Link